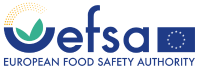
Bactrocera dorsalis
European Food Safety Authority (EFSA), Melanie Camilleri
Updated 12 December 2022 ( Version 3 )
Abstract
This document is an update of the pest survey card on Bactrocera dorsalis that was prepared in the context of the EFSA mandate on plant pest surveillance (M-2017-0137) at the request of the European Commission. Its purpose is to guide the Member States in preparing data and information for surveys for Bactrocera dorsalis (Hendel, 1912) (Diptera: Tephritidae). These are required to design statistically sound and risk-based pest surveys in line with current international standards. Bactrocera dorsalis belongs to a group of closely related species that are recognised as the B. dorsalis species complex. Non-European Tephritidae, including B. dorsalis, are regulated as Union quarantine pests. Bactrocera dorsalis is also listed as a priority pest. It is endemic and widespread in tropical areas of Southeast Asia, and it has also been introduced to Africa where it is now spread almost throughout the entire sub-Saharan region. Isolated detections in the EU are considered to be linked to the import of infested exotic fruits, but in 2022 an outbreak has been reported in the South of Italy. Bactrocera dorsalis has a wide host range of fruit crops. The primary host crops for targeted surveillance in the EU should be Citrus species, Prunus species, Persea americana (avocado) and Mangifera indica (mango). Climatic models show that the southernmost regions in the EU may have ecoclimatic conditions that are suitable for the establishment of B. dorsalis. The import and movement of infested fruit is considered the most likely pathway for introduction of B. dorsalis. Trapping is the recommended method for surveillance and detection and species identification should be performed by a taxonomist.
© European Food Safety Authority, 2022
Heading picture: Florida Division of Plant Industry, Florida Department of Agriculture and Consumer Services, Bugwood.org, UGA5193076.
Authors' affiliation: Melanie Camilleri, European Food Safety Authority.
Reproduction is authorised provided the source is acknowledged with the exception of the images where the copyright is indicated, for which reproduction is prohibited and permission must be sought directly from the copyright holder.
Introduction
The objective of this pest survey card is to provide the relevant information needed to prepare surveys for B. dorsalis in EU Member States (MSs) following the methodology described in EFSA et al. (2018) . It is part of a toolkit that has been developed to assist the MSs with planning a statistically sound and risk-based pest survey approach in line with the recommendations and guidelines provided by the International Plant Protection Convention (IPPC) in the various International Standards for Phytosanitary Measures ( ISPM 6: FAO, 2021a ; ISPM 31: FAO, 2021b) and surveillance guide ( FAO, 2021c) . The EFSA Plant Pest Survey Toolkit consists of pest specific documents and more general documents relevant for all pests to be surveyed:
i. Pest-specific documents:
a. The pest survey card on Bactrocera dorsalis
ii. General documents:
a. General guidelines for statistically sound and risk-based surveys of plant pests (EFSA et al., 2020)
b. The statistical tools RiBESS+ and SAMPELATOR.
c. The RiBESS+ manual and video tutorial .
This document is an update of the pest survey card on B. dorsalis (EFSA, 2020) that was prepared in the context of the EFSA mandate on plant pest surveillance (M-2020-0114) at the request of the European Commission. The information presented in this pest survey card was summarised from a pest report by EFSA (2019) , the International Plant Protection Convention (IPPC) protocol on Bactrocera dorsalis (FAO, 2019), the European and Mediterranean Plant Protection Organization (EPPO) Global Database, the Centre for Agriculture and Bioscience International (CABI) Invasive Species Compendium (CABI, 2019), and reviewed literature.
The main challenge relevant for surveillance of B. dorsalis are related to the incomplete information on host range and the diagnostic tools that rely mostly on morphological identification by a specialist taxonomist on adult flies.
1. The pest and its biology
1.1. Taxonomy
Current scientific name: Bactrocera dorsalis (Hendel) Class: Insecta Order: Diptera Family: Tephritidae Genus: Bactrocera Species: Bactrocera dorsalis Synonyms: among others Bactrocera ferruginea Bezzi, 1913; Bactrocera invadens Drew, Tsuruta & White, 2005; Bactrocera papayae Drew & Hancock, 1994; Bactrocera philippinensis Drew & Hancock, 1994; Bactrocera (Bactrocera) variabilis Lin & Wang, 2011; Chaetodacus ferrugineus Bezzi, 1916; Chaetodacus ferrugineus var. dorsalis Hendel, 1915; Chaetodacus ferrugineus dorsalis Bezzi, 1916; Chaetodacus ferrugineus var. okinawanus Shiraki, 1933; Dacus ferrugineus (Fabricius, 1805); Dacus dorsalis Hendel, 1912; Dacus (Bactrocera) semifemoralis Tseng, Chen & Chu, 1992; Dacus (Bactrocera) yilanensis Tseng, Chen & Chu, 1992; Musca ferruginea Fabricius, 1794; Strumeta dorsalis Hering, 1956; Strumeta ferruginea Hering, 1956; Strumeta dorsalis okinawana Shiraki, 1968. EPPO Code: DACUDO Common name: Oriental fruit fly Taxonomic rank: species
Bactrocera dorsalis (Hendel), the objective of this card, is part of a group of closely related species that are recognised as a B. dorsalis complex (Drew and Hancock, 1994; Drew, 2004; Clarke et al., 2005). Species in the complex have very similar morphology and require identification by a taxonomist, but this similarity is based on convergent characters and the complex does not represent a monophyletic lineage (Leblanc et al., 2015). The complex is comprised of over 75 species (Augustinos et al., 2014; Schutze et al., 2015b; Schutze et al., 2017b; EFSA PLH Panel, 2020 ), which includes significant agricultural pests (White and Elson-Harris, 1992; Vargas et al., 2015), including B. dorsalis (Hendel) which is a polyphagous pest causing fruit loss in several commercially grown crops (Vargas et al., 2015).
The taxonomy of species in the B. dorsalis complex is uncertain; and hybridization within the complex has been reported (Doorenweerd et al., 2018), thus adding doubts on the inclusion of some taxa such as B.carambolae (e.g. Vaníčková et al., 201; EFSA PLH Panel, 2020 ).
This pest survey card on B. dorsalis includes former species B. invadens, B. papayae and B. philippinensis, which are now considered synonyms of B. dorsalis (Hendel).
1.2. EU pest regulatory status
Non-European Tephritidae, including B. dorsalis, are regulated as Union quarantine pests in Annex II, Part A of Commission Implementing Regulation (EU) 2019/2072 . This part of the Annex contains pests not known to occur in the Union territory.
Special requirements for the introduction into the Union territory of fruits of Citrus L., Fortunella Swingle, Poncirus Raf., and their hybrids, Mangifera L. and Prunus L., originating from third countries, are laid down in Annex VII of the same Implementing Regulation.
Bactrocera dorsalis is also listed as a priority pest under Commission Delegated Regulation (EU) 2019/1702 , for which annual surveys are required.
The general requirements for survey of quarantine pests in the EU territory are laid down in Regulation (EU) 2016/2031 and Commission Implementing Regulation (EU) 2020/1231 .
1.3. Pest distribution
Bactrocera dorsalis is endemic and widespread in tropical areas of Southeast Asia (Clarke et al., 2005). Almost all the other species of the B. dorsalis complex mainly occur in the same region. The distribution of B. dorsalis in Southeast Asia has not changed during the 20th and 21st centuries (Clarke et al., 2019), other than the northward invasion of B. dorsalis into central China, which is most likely linked to fruit trade (Qin et al., 2018). In the Pacific, B. dorsalis has also been introduced to the Northern Mariana Islands (1935), Hawaii (1945), Guam (1947, eradicated), and French Polynesia (1996) (EFSA, 2019). The species has also been introduced to Africa and since its first appearance (initially described as B. invadens) in Kenya in 2003, B. dorsalis has spread almost throughout the entire sub-Saharan region (Lux et al., 2003; Goergen et al., 2011). In Africa, the species mainly prevails in warm humid lowland, cultivated and forested areas, and has displaced other tephritid species (Ekesi et al., 2006; Rwomushana et al., 2008a). In the USA, repeated captures in Florida and almost annual captures in California have triggered a recurrent eradication programme (Papadopoulos et al., 2013; FDACS, 2018). The pest was reported to be eradicated in Florida in 2016. Populations in California could be self-sustaining and thus have become established (Papadopoulos et al., 2013), but this claim is challenged by others (Shelly et al., 2017). In the United States B. dorsalis is present only in one area in California where is under official control (NAPPO, 2021). Transient populations of B. dorsalis have been detected several times in Torres Strait islands, Australia, likely originated from individuals flying from New Guinea, butthey are recurrently eradicated (FAO, 2021d).
In 2016, one adult of B. dorsalis species complex was caught in a fruit market in Vienna, Austria, during a national survey (Egartner and Lethmayer, 2017; Egartner et al., 2018). Additional individuals were trapped in Vienna between 2012 and 2018, but all findings occurred in urban areas and considered to be linked to repeated entries of larvae in infested fruit and not to an outbreak. In France, the insect was trapped in the Ile-de-France region, near Paris and in Provence-Alpes-Côte d'Azur from 2019 to 2021, and additional isolated finding were reported in the Alsace region close to a point of entry in 2022 ( EPPO, online-b ). All these findings were linked to the import of infested exotic fruits, and not to outbreaks. Isolated findings of B. dorsalis were detected in Campania and Latium regions in Italy starting from November 2018 ( EPPO, online-a ; Nugnes et al., 2018). In 2022, a demarcated area including four municipalities and a buffer zone of 7.5 km were established in the Naples province after hundreds of specimens trapped in just few months ( EPPO, 2022 ).
Note: the information included in this section is aligned with the EPPO map updated on 03-11-2022
1.4. Life cycle
Bactrocera dorsalis females lay white to creamy-yellow eggs under the fruit skin, which exhibit discoloration around the oviposition puncture. (Rwomushana et al., 2008b, Danjuma et al., 2014; Salum et al., 2014). Mature larvae emerge from the fruit, drop to the ground and pupate within a tan to dark brown puparium in the soil (Weems et al., 1999) (figure in the right panel). Adult flies emerge from the soil and require about nine days to reach sexual maturity, but this period is considerably longer when temperatures are cool (Weems et al., 999). A single female produces about 1,200 to 1,500 eggs during her lifetime. Flies prefer ripe fruit for oviposition, but unripe fruits may also be attacked (Weems et al., 1999). Bactrocera dorsalis is multivoltine, completing several overlapping generations every year and populations may rapidly increase in number (Stephens et al., 2007; Theron et al., 2017). The development time of B. dorsalis from egg to adult stage, ranges from 16 days at 30 °C to 76 days at 15 °C in laboratory conditions (Rwomushana et al., 2008; Dongmo et al., 2021).
2. Target population
This section provides the information needed to characterise the population of host plants to target in a survey, as described in the ‘General guidelines for statistically sound and risk-based surveys of plant pests’ (EFSA et al., 2020). This includes the pest’s host range and main hosts in the EU ( Section 2.1 ), the suitability of EU environments to the pest’s establishment ( Section 2.2 ), the ability of the pest to spread ( Section 2.3 ), and the identification of risk factors associated with an increased probability of presence ( Section 2.4 ).
Once the above parameters have been defined, the target population can be structured in multiple levels. At level 1 is the survey area, which corresponds to the entirety or part of the Member State. At levels 2 and 3 are the epidemiological units that can be distinguished within the survey area. Epidemiological units can be chosen as administrative regions (e.g. EU NUTS areas or Member State-level regions) if they are homogeneous, or further subdivided into the environments where host plants are present using a land-use categorisation (e.g. urban, agricultural and natural areas, nurseries). At level 4, if risk factors are identified, the risk areas are defined around the risk locations. At level 5 are the inspection units, the elementary subdivisions of the target population that are inspected for the detection of the pest (e.g. host plants), depending on the pest detection method ( Section 3 ). For the definitions of the target population, epidemiological units and inspection units, see also the glossary of terms available at the end of this document.
The hierarchical structure of the target population should be tailored to the situation in each Member State. A possible structure of the target population for surveys of Bactrocera dorsalis within the EU is proposed in Section 2.5 .
2.1. Host range and main hosts
Bactrocera dorsalis is highly polyphagous and can cause damage to a wide range of fruit crops. It is not possible to define the exact host range because the species can easily be confused with related species, several of which have recently been described and whose taxonomic status continues to be debated. Hence, records which indicate that a particular crop is a host may concern other species within the B. dorsalis species complex. Altogether, species in the B. dorsalis complex attack over 270 plant species (Vargas et al., 2015). The information on host-range is still incomplete and when presented with ‘new’ plant species the fly is often able to utilize them, such as the case of Sclerocarya birrea (marula) and Sorindeia madagascariensis (Mtikiza) in Africa (Rwomushana et al., 2008b). In addition, studies targeting wild hosts are underrepresented relative to commercial crops.
Studies reporting infestation of particular host are numerous, often indicating that B. dorsalis is a major pest. However, quantification of infestation rates and economic impact are rarely included in these studies. Clarke et al. (2005) summarised infestation of fruit of economic importance in its native range in Southeast Asia; among the host plants are many tropical species: Annona spp. (custard apple), Artocarpus altilis (breadfruit), A. heterophyllus (jackfruit), Averrhoa carambola (carambola), Carica papaya (papaya), Chrysophyllum spp. (star apple), Dimocarpus longan (longan), Garcinia mangostana (mangosteen), Litchi chinensis (lychee), M. indica (mango), Manilkara zapota (sapodilla), Musa spp. (banana and plantain), Nephelium lappaceum (rambutan), Passiflora edulis (passion fruit), Pouteria sapota (sapote), Psidium guajava (guava). In addition, Clarke et al. (2005) lists several hosts that are grown in Southeast Asia, but are also of economic importance in the EU: Capsicum spp., Citrus spp. (including orange, lemon, and lime), Cucumis melo (melon), Cucumis sativus (cucumber), Solanum lycopersicum (tomato), Malus domestica (apple), Persea americana (avocado), Prunus avium (sour cherry), P. domestica (plum), P. persica (peach and nectarine) and Solanum melongena (eggplant). The tropical host species are mainly of relevance when determining the risk locations (import, packaging stations), while the second list of hosts is relevant for both selecting risk locations and hosts to be surveyed.
Outside its native range, host preference studies on B. dorsalis (formerly B. invadens) have been conducted, for example, in Kenya (Rwomushana et al., 2008b). Mangifera indica (mango), Musa spp. (bananas and plantains), Citrus spp. (lime, mandarin and sweet orange) are reported to be among the cultivated species heavily infested. In Hawaii, Citrus spp. (tangerine, orange, grapefruit, lemon), Ficus carica (fig), P. guajava (guava), Eriobotrya japonica (loquat), M. indica (mango), Momordica balsamina (momordica), Prunus persica (peach and nectarine), Diospyros (persimmon), Feijoa sellowiana (pineapple guava), Prunus domestica (plum), Syzygium jambos (malabar plum), Psidium cattleianum (strawberry guava), M. zapota (sapodillia) and Eugenia uniflora (Surinam-cherry) are regular hosts of B. dorsalis (Vargas et al., 2010a). Especially, the various Citrus species, P. persica (peach and nectarine), Syzygium jambos (rose apple), E. japonica (loquat) and P. guajava (guava) were frequently infested in Hawaii.
Based on the information in EFSA (2019) above and the EPPO Global Database (online) and CABI (2019), the primary host crops for targeted surveillance in the EU would be the various Citrus species, Prunus species (particularly P. persica (peach and nectarine)), Persea americana (avocado) and M. indica (mango). To date, the main production areas of the latter two host fruits are located in Spain.
Han et al. (2011) reported B. dorsalis on pome fruit, therefore pear and apple could also be targeted in the surveillance. Further details are presented in Section 2.2 .
2.2. Environmental suitability
Bactrocera dorsalis is essentially a tropical species, but climatic models show that the southernmost regions in the EU may have ecoclimatic conditions that are suitable for the establishment of B. dorsalis (Stephens et al., 2007; De Villiers et al., 2016; EFSA, 2019 ). Temperature, relative humidity and rainfall affect the survival, flight activity and population dynamics of B. dorsalis. The exact effects of temperature and moisture may vary depending on vegetation type, rainfall patterns and agricultural practices (Adzim et al., 2016), but in general the species performs best in moist and high temperature conditions (Adzim et al., 2016; Rwomushana et al., 2008b). According to Fletcher (1987), temperatures outside the optimum range of 18°C to 27°C increase mortality until the point that no individuals survive long enough to complete development. However, the species can also tolerate climates with drier winters (CABI, 2019). According to Stephens et al. (2007) there is currently a marginal risk for B. dorsalis to become established in warm Mediterranean climates, but climate change is predicted to expand the potential area of distribution northwards to much of Spain, Portugal, Italy and the south of France. De Villiers et al. (2016) reviewed previous attempts to model the distribution of B. dorsalis and published their own projection of global potential distribution using the CLIMEX species distribution model with irrigation scenarios and climate change scenarios. EFSA (2019) subsequently used the baseline projection of De Villiers et al. (2016) and a composite irrigation scenario combined with the Joint Research Centre (JRC) weather station data from the time period 1990–2007 to define the area of potential establishment of B. dorsalis. Under this scenario, B. dorsalis would be able to become established in the Mediterranean coastal areas of southern Spain, Portugal, Italy, Croatia, Slovenia, Greece and Cyprus, and parts of the Atlantic coast in France.
Other than temperature and humidity, B. dorsalis needs a continuous availability of fruits, in order to maintain a viable population. In the tropical regions in which the species is currently distributed, such a continuous supply would be available. In the Mediterranean area, the availability of citrus fruit from September to June, and subsequent presence of several other host species from May to September ( EPPO, 2009 ) also ensures that a continuous supply of fresh fruit is available. Further north in the EU, host fruit availability may become a limiting factor for establishment.
The risk of glasshouses in northern Europe becoming ‘infested’ with B. dorsalis (B. invadens) is considered very unlikely ( EPPO, 2009 ). In China, the Hubei province has a subtropical climate with hot and wet summers and cold winters. Bactrocera dorsalis was first reported there in 2004 (Han et al., 2011) and, because of the cold winter, the population dynamics in this area are highly relevant for assessing establishment and spread into the EU. The fly could complete four to five generations from July to December, shifting to hosts such as pear, jujube, persimmon and sweet orange. Adults and larvae cannot survive the cold period in the winter, but some pupae could survive when placed in the soil (Han et al., 2011). A small proportion of overwintering pupae may lead to a small initial number of adults early in the season and ensure survival of the population. This survival likely constitutes a strong selection pressure for better cold tolerance in B. dorsalis and future expansion of its distribution range.
2.3. Spread capacity
Natural spread
Bactrocera dorsalis is considered a strong flier. Immature adults can disperse over long distances to find fresh food resources and breeding substrates (Fletcher, 1987; Steiner, 1957). Large numbers of flies usually move into fruiting areas when fruit begins to ripen, and they may leave when the fruiting season ends. As long as sufficient food sources are available in the nearby surroundings one would expect limited dispersal, so that flies remain in the area where they emerged to lay the next generation of eggs. Based on an expert knowledge elicitation, with regards to the spread rate, EFSA (2019) estimated that the maximum distance expected to be covered in one year by B. dorsalis is 7 km (with a 95% uncertainty range of 1.4 – 34km). This scenario considers 2–3 generations per year with 3–4 km spread per generation. For instance, in a mark-and-recapture experiment in Hawaii, 30 adults were captured at distances of over 2 km, ranging from 2.63 to 11.39 km (Froerer et al., 2010). Steiner (1957) found a dispersal radius of B. dorsalis of up to 37 km after fruit harvests in Hawaii, and Zhu and Qiu (1989) reported that B. dorsalis could cover the 27 km distance from Taiwan to Ryukyu.
Human-assisted spread
The most likely introduction of B. dorsalis would be via transport of infested fruit (imports of fruit commodities or fruit in passenger luggage) because the development of eggs and larvae takes place inside the fruit which makes the infestation difficult to discover. According to the EUROPHYT database, several interceptions of B. dorsalis from countries where the pest is present are reported in Europe annually in consignments of infested fruit (mainly on mango). Although the event of entry of B. dorsalis is more likely to happen in early life stages with infested fruit, the majority of cases of entry will not result in outbreaks. The likelihood of importing adults can be considered negligible. When eggs or larvae survive the transport, larvae still need to pupate and then reach the adult stage. The adult female flies subsequently need to locate both a suitable mate and a suitable host plant with fresh fruit in order to lay eggs for the next generation of flies.
The additional possibility of having pupae in the soil or other growing medium with the host plants should not be excluded.
2.4. Risk factor identification
Identification of risk factors and their relative risk estimation are essential for performing risk-based surveys. A risk factor is a biotic or abiotic factor that increases the probability of infestation by the pest in the area of interest. The risk factors that are relevant for surveillance need to be characterised by their relative risk (should have more than one level of risk for the target population) and the proportion of the overall target population on which they apply. The identification of risk factors needs to be tailored to the situation of each Member State. This section presents examples of risk factors for B. dorsalis and is not necessarily exhaustive.
For the identification of risk areas, it is first necessary to identify the activities that could contribute to introduction or spread of B. dorsalis. These activities should then be connected to specific locations. Around these locations, risk areas can be defined, knowing that their size depends on the spread capacity of the target pest and the availability of host plants around these locations.
The Member States can opt to utilise the information available on the EU Platforms of TRACES Interceptions, EUROPHYT Interceptions and EUROPHYT Outbreaks. The information available, in particular, relating to the country of origin, type of commodity and hosts of intercepted or outbreak reports can be extracted from such platforms for specific harmful organisms. This information can allow Member States to identify potential pathways of introduction from previous historical findings. Thus, Member States might consider focusing their surveillance efforts around activities and locations related to previous interceptions and outbreaks.
Such information should only be considered as indicative and given the possible dynamic changes, it should be reviewed and analysed periodically.
Example 1: Import locations and packing stations
Given that import and movement of infested fruit constitute the most likely pathway for introduction, activities such as import locations, packing stations, production sites, fresh fruit markets and processing industries that handle this fruit, would be locations with a higher probability of finding the pest. This is particularly true when the fruit originate from areas where B. dorsalis is present and when this fruit is a regular host for this species in those areas. Activities dealing with both the tropical host species and hosts of economic importance in the EU such as Citrus spp., Prunus spp., avocado, mango and pome fruits, could be considered as risk locations. The vicinity of the import locations and packing stations could be considered to be risk areas. The host species of economic importance in the EU (such as Citrus spp. or Prunus spp.) present in risk areas could be selected as the primary host crops for surveys, for example, orchards.
Given that B. dorsalis is a strong flier with a good capacity for natural spread ( Section 2.3 ), the risk area will be of considerable size. The relative risk of the specific area will depend on the nature of the activities that are taking place at the high-risk location, e.g. the timing and origin of the import, type of fruit, the method of fruit processing, use of cooling facilities, etc. The table on the right, shows some examples of risk activities and corresponding risk locations relevant for surveillance of B. dorsalis.
2.5. Structure of the target population
The figure on the right panel gives examples of the components of a target population for B. dorsalis and is not necessarily exhaustive.
3. Detection and identification
Bactrocera dorsalis can be detected by trapping adults using attractant lures or by examining fruits for the presence of larvae or signs of oviposition. Trapping would be the recommended method for surveillance, and when examinations of fruits are already performed to detect other pests, it might be worthwhile to include B. dorsalis in the respective protocol.
3.1. Detection and identification in the field
3.1.1. Visual examination
Pest
The morphological identification of B. dorsalis should be performed by an expert taxonomist. Morphological identification of eggs, larvae or pupae to the B. dorsalis species level is not reliable because the immature stages from different Bactrocera species are too similar.
A detailed description of the eggs, larvae and pupae can be found in, e.g. Drew and Romig (2016) and White and Elson-Harris (1992), while simplified descriptions are available in the CABI datasheet on B. dorsalis (CABI, 2019) or in Weems et al. (1999).
Adult morphology exhibits great variation among populations of B. dorsalis. The body length of the adults is about 8.0 mm and the mostly hyaline wings are approximately 7.3 mm in length (Weems et al., 1999). Body colour is variable, but prominent yellow and dark brown to black markings are always present on the thorax. Generally, two horizontal black stripes are present on the abdomen with a longitudinal median stripe extending from the base of the third segment to the apex. These stripes form a T-shaped pattern, but the pattern varies considerably. The ovipositor is very slender and sharply pointed (Weems et al., 1999).
Signs and symptoms
In general, fruit can be examined for puncture marks in its skin, produced by female during oviposition. Puncture marks can be recognised by discolouration of the fruit skin, dark spots, and sometimes by fruit juice exuding from the puncture hole. The fruit infested with eggs or larvae have symptoms of oviposition stings on the surface, e.g. discolouration and a slight depression in the fruit surface. Oviposition stings vary greatly depending on the type of fruit making detection more difficult ( EFSA PLH Panel, 2020 ).
Eggs might be found inside the fruit at the point of the oviposition puncture, but such punctures would initially be difficult to detect unless examined under a microscope and by an expert (FAO, 2019). At a more advanced stage, the area around the puncture marks becomes soft (larval feeding causes the fruit structure to disintegrate).
The collection of potentially infested fruits with larvae can also be considered as a method of detection for B. dorsalis. Larvae can be detected when opening the fruit, especially once they have become advanced third instars. Larvae can be reared to the adult stage for identification ( Section 3.2.2 ).
3.1.2. Trapping
Male flies of the genus Bactrocera are detected mainly by lured adult traps. Guidance on trapping Bactrocera fruit flies is given in Appendix 1 of ISPM 26 (FAO, 2018). Male flies of B. dorsalis are strongly attracted to methyl eugenol (4-allyl-1, 2-dimethoxybenzene-carboxylate). The efficacy of this lure has been proven in numerous studies. Methyl eugenol also attracts several other species of the genus Bactrocera, including B. carambolae, B. caryeae, B. correcta, B. kandiensis, B. musae, B. occipitalis (FAO, 018). The non-native – but established – B. oleae is not attracted by methyl eugenol and is attracted by the pheromone spiroketal instead (FAO, 2018). Baiting with methyl eugenol is also applied as a male annihilation technique for field suppression eradication or of B.dorsalis (Koyama et al., 1984; Manoukis et al., 2017; Vargas et al., 2010b).
A wide variety of systems are available to trap B. dorsalis and other tephritid species. Various trapping systems are being used in combination with methyl eugenol (e.g. McPhail-type traps in Manrakhan, 2016; Lynfield traps in Odanga et al., 2018; Steiner traps in Kumar and Agarwal, 2005; Jackson traps in Shelly et al., 2004; yellow sticky traps in Manoukis et al., 2017; mineral water bottle traps and the yellow-top plastic traps in Adzim et al., 2016; and bucket-type Moroccan traps in Manrakhan, 2016). Methyl eugenol is highly volatile and can be applied on a cotton wick, which is then placed on the bottom or inside the trap (depending on the type of trap). The eastern side of the tree, which receives sunlight in the early hours of the day and resting and feeding areas in plants that provide shelter and protect fruit flies from strong winds and predators would be a suitable trap site (FAO, 2018).
Samples from the traps should be collected at regular intervals and taken to the laboratory for further confirmation, and lures should be replaced at regular intervals.
Food-based attractants can be used for trapping both male and female flies and can be composed of synthetic volatile components of hydrolysed protein substances or the hydrolysed protein substances themselves. Food-based attractants are generic by nature, and traps with food-based attractants tend to catch a wide range of other non-target tephritids and non-tephritid fruit flies next to the target species. This could be beneficial when targeting multiple species at the same time but could also result in overlooking the target species when non-target species (E.g. Ceratitis capitata) dominate the catch. In general, male lures are preferred over food-based attractants in early detection surveys given the higher specificity and longer range of attraction. However, female targeted attractants may be more sensitive to detect low adult populations of fruit flies in the wild (Papadopoulos et al., 2001).
Based on measured distance-dependent capture probabilities for B. dorsalis males in southern California, Shelly et al. (2010) estimated that populations with as few as about 50 males would always (p > 0.999) be detected using a trap density of five methyl eugenol-baited traps per square mile (one trap per 50 hectares). Appendix of ISPM 26 also gives guidance on trapping densities (FAO, 018), indicating that a single trap per square kilometre suffices when performing a detection survey in a production area within a pest-free area to verify the absence of Bactrocera spp. responding to methyl eugenol.
Additional information on the commonly used trapping devices can be found in the table from the EFSA pest categorisation of non-EU Tephritidae (Table 2, EFSA PLH Panel, 2020 ).
3.1.3. Sample collection
Any potentially infested fruit should be collected, e.g. in sealed bags, and taken to the lab for further confirmation. If collected larvae are to be preserved, they should be placed in boiling water for a few seconds. Once they become immobile, they can either be transferred to 70% ethanol (for morphological identification at the genus level) or to 95% ethanol (for molecular tests to support identification) ( EPPO, 2018 ).
3.1.4. Timing of detection and identification
The optimal timing for survey activities depends on the host fruiting period. The presence of ripe fruits determines the possibility to trap emerging adults and to find larvae within fruits. This should be assessed for the various targeted host plants in each Member State.
3.2. Detection and identification in the laboratory
3.2.1. Morphological identification
Accurate identification is important for specimens intercepted during import inspections and for those detected, for example, in traps ( EFSA PLH Panel, 2020 ).
Identification of B. dorsalis at the species level requires morphological examination of adult flies, as is generally the case for Tephritidae. It is not possible to identify a fly to the B. dorsalis complex using immature life stages, let alone identify B. dorsalis to the species level (FAO, 2019).
Keys for the morphological identification of the family Tephritidae are available. The FAO protocol (2019) for adult B. dorsalis fruit flies also includes five other species of the B. dorsalis complex of economic importance that can be confused with B. dorsalis, namely B. carambolae, B. caryeae, B. kandiensis, B. occipitalis and B. pyrifoliae. In the FAO protocol, the former species B. invadens, B. philippinensis and B. papaya are considered as a part of B. dorsalis sensu lato.
3.2.2. Laboratory testing and other methods of identification
As reviewed in EFSA PLH Panel (2020) B. carambolae can be distinguished from B. dorsalis using existing molecular and chemical markers.
A protocol for DNA barcoding based on the cytochrome oxidase I (COI) gene is described in PM 7/129 on DNA barcoding as an identification tool for a number of regulated pests ( EPPO, 2016 ) and can be used for all life stages. This protocol can provide additional information to support morphological identification of B. dorsalis. However, molecular identification by DNA sequencing of the COI gene does not provide sufficient resolution to identify many species in the B. dorsalis complex.
Larvae can be reared to the adult stage for identification and this is required to accurately identify B. dorsalis or other Bactrocera species. According to the FAO (2019) protocol, ‘larvae can be reared to adults by placing infested fruits in cages containing a pupation medium (e.g. damp vermiculite, sand or sawdust) at the bottom. The cages are covered with cloth or fine mesh. Once the larvae emerge from the fruit, they will move to the pupation medium. Each sample should be observed, and pupae gathered daily. The pupae are placed in containers with the pupation medium, and the containers are covered with a tight lid that enables proper ventilation. Once the adults emerge, they must be kept alive for several days to ensure that the tegument and wings acquire the rigidity and characteristic coloration of the species. Flies can be fed with honey (sugar) and water. The adults are then killed by freezing, or by exposure to ethyl acetate or other killing agents appropriate for morphological examination, and then mounted on pins. Prior to mounting (before they harden), it is useful to gently squeeze the apical part of the pre abdomen with forceps, then squeeze the base and apex of the oviscape to expose the aculeus tip for females, and to pull out the aedeagus for males. Alternatively, this will need to be dissected later in flies.
4. Conclusion
Information on what, where, when and how to conduct survey activities or B. dorsalis is summarised in the Table shown. The identification of the target population needs to be tailored to the situation in each Member State (example shown below).
5. Survey framework
The figure below shows the next steps after the survey preparation for designing statistically sound and risk-based detection and delimiting surveys of B. dorsalis. Guidance on selecting the type of survey, related survey preparation and design, is provided in the the EFSA general guidelines for pest surveys on the right panel (EFSA et al., 2020).
Glossary
Scroll down the right panel to access the definitions included in the glossary.
Relevant EFSA outputs
Acknowledgments
EFSA wishes to acknowledge Antoon Loomans and Martijn Schenk of the Netherlands Food and Consumer Product Safety Authority (NVWA) in the context of the grant GP/EFSA/ALPHA/2017/02 for the preparation, Nikolaos Papadopoulos for the review, and EFSA staff Mart Kinkar and Sybren Vos and EFSA trainee Makrina Diakaki for finalisation and publication of the first version of the survey card on Bactrocera dorsalis (EFSA-Q-2019-00276).
EFSA wishes to thank Maria Chiara Rosace for the preparation of the first version of the survey card in story map format in the context of the EFSA procurement PO/EFSA/ALPHA/2020/05.
EFSA also wishes to thank EFSA staff Melanie Camilleri for the preparation and publication of this update of the pest survey card on Bactrocera dorsalis (EFSA-Q-2022-00136).
Suggested citation: EFSA (European Food Safety Authority), 2022. Pest survey card on Bactrocera dorsalis. EFSA supporting publication 2022:EN-7206. Available online: https://efsa.europa.eu/plants/planthealth/monitoring/surveillance/bactrocera-dorsalis . Last updated: 12 December 2022.
Contact us: PLANTS@efsa.europa.eu
References
Adzim CA, Billah MK and Afreh-Nuamah K, 2016. Abundance of African invader fly, Bactrocera invadens drew, tsuruta and white (diptera: tephritidae) and influence of weather parameters on trap catches in mango in the Volta region of Ghana. Springer Plus, 5, 968.
Augustinos AA, Drosopoulou E, Gariou-Papalexiou A, Bourtzis K, Mavragani-Tsipidou P and Zacharopoulou A, 2014. The Bactrocera dorsalis species complex: comparative cytogenetic analysis in support of Sterile Insect Technique applications. BMC Genet 15, S16. https://doi.org/10.1186/1471-2156-15-S2-S16
Bhagat D, Samanta SK and Bhattacharya S, 2013. Efficient Management of Fruit Pests by Pheromone Nanogels. Scientific Reports, 3, 1294.
CABI (Centre for Agriculture and Bioscience International), 2019. Invasive Species Compendium. Datasheet Bactrocera dorsalis (Oriental fruit fly). 9/7/19. Wallingford, UK: CAB International. Available online: https://www.cabi.org/isc/datasheet/17685 . Accessed 17 July 2019.
Clarke AR, Armstrong KF, Carmichael AE, Milne JR, Raghu S, Roderick GK and Yeates DK, 2005. Invasive phytophagous pests arising through a recent tropical evolutionary radiation: the Bactrocera dorsalis complex of fruit flies. Annual Review of Entomology, 50, 293–319.
Clarke AR, Li ZH, Qin YJ, Zhao ZH, Liu LJ and Schutze MK, 2019. Bactrocera dorsalis (Hendel) (Diptera: Tephritidae) is not invasive through Asia: It's been there all along. Journal of Applied Entomology, in press.
Danjuma S, Thaochan N, Permkam S and Satasook C, 2014. Effect of temperature on the development and survival of immature stages of the carambola fruit fly, Bactrocera carambolae, and the Asian papaya fruit fly, Bactrocera papayae, reared on guava diet. Journal of Insect Science, 14, 126.
De Villiers M, Hattingh V, Kriticos DJ, Brunel S, Vayssières J-F, Sinzogan A, Billah M, Mohamed S, Mwatawala M and Abdelgader H, 2016. The potential distribution of Bactrocera dorsalis: considering phenology and irrigation patterns. Bulletin of Entomological Research, 106, 19–33.
Dongmo K Michel A, Komi Fiaboe K M, Sévilor Kekeunou, Samuel Nanga N, Apollin Kuate F, Henri Tonnang E Z, Désiré Gnanvossou, Rachid Hanna, 2021. Temperature-based phenology model to predict the development, survival, and reproduction of the oriental fruit fly Bactrocera dorsalis. Journal of Thermal Biology, Volume 97.
Doorenweerd C, Leblanc L, Norrbom AL, San Jose M and Rubinoff R, 2018. A global checklist of the 932 fruit fly species in the tribe Dacini (Diptera, Tephritidae). ZooKeys, 730, 17–54.
Drew RAI, 2004. Biogeography and Speciation in the Dacini (Diptera: Tephritidae: Dacinae). In: Evenhuis NL, Kaneshiro KY (Eds). D. Elmo Hardy Memorial Volume. Contributions to the Systematics and Evolution of Diptera, Bishop Museum, Honolulu, HI, 165–178.
Drew RAI and Hancock DL, 1994. The Bactrocera dorsalis complex of fruit flies (Diptera: Tephritidae: Dacinae) in Asia. Bulletin of Entomological Research, Supplement Series 2, 1–68.
Drew RAI and Romig MC, 2016. Keys to the tropical fruit flies (Tephritidae: Dacinae) of south-east Asia. Wallingford, UK, CABI. 496 pp.
Ekesi S, Nderitu P and Rwomushana I, 2006. Field infestation, life history and demographic parameters of the fruit fly Bactrocera invadens (Diptera: Tephritidae) in Africa. Bulletin of Entomological Research, 96, 79–386.
EFSA (European Food Safety Authority), 2018. Technical report of the methodology and work-plan for developing plant pest survey guidelines. EFSA supporting publication 2018: EN-1399. 36 pp. doi:10.2903/sp.efsa.2018.EN-1399 .
EFSA, Baker Richard, Gilioli Gianni, Behring Carsten, Candiani Denise, Gogin Andrey, Kaluski Tomasz, Kinkar Mart, Mosbach-Schulz Olaf, Neri Franco Maria, Preti Stefano, Rosace Maria Chiara, Siligato Riccardo, Stancanelli Giuseppe and Tramontini Sara, 2019. Bactrocera dorsalis - Pest Report and Datasheet to support ranking of EU candidate priority pests [Data set]. Zenodo. http://doi.org/10.5281/zenodo.2786922
EFSA (European Food Safety Authority), 2020. Story map for survey of Bactrocera dorsalis. EFSA supporting publication 2020:EN-1861. Available online: https://arcg.is/0aXqST . Last updated: 5 May 2020.
EFSA PLH Panel (EFSA Panel on Plant Health), Bragard C, Dehnen-Schmutz K,Di Serio F, Gonthier P, Jacques M-A, Jaques Miret JA, Justesen AF, Magnusson CS, Milonas P, Navas-Cortes JA, Parnell S, Potting R, Reignault PL, Thulke H-H, Van der Werf W, Vicent Civera A, Yuen J, Zappalà L, Bali EM, Papadopoulos N, Papanastassiou S, Czwienc zek E and MacLeod A, 2020. Pest categorisation of non-EU Tephritidae. EFSA Journal 2020;18(1):5931, 62 pp. https://doi.or g/10.2903/j.efsa.2020.5931
Egartner A and Lethmayer C, 2017. Invasive fruit flies of economic importance in Austria—Monitoring activities. Integrated Protection of Fruit Crops, IOBC-WPRS Bulletin, 123, 45–49.
Egartner A, Lethmayer C and Blümel S, 2018. Monitoring activities on invasive fruit flies (Tephritidae, Diptera) in Austria. In: Proceedings of the XI European Congress of Entomology, Naples, Italy, 2–6 July p. 153.
EPPO (European and Mediterranean Plant Protection Organization), online-a. EPPO Global Database - Bactrocera dorsalis (DACUDO). Distribution details in Italy. Available online: https://gd.eppo.int/taxon/DACUDO/distribution/IT [Accessed: 05 December 2022]
EPPO (European and Mediterranean Plant Protection Organization), online-b. EPPO Global Database - Bactrocera dorsalis (DACUDO). Distribution details in France. Available online: https://gd.eppo.int/taxon/DACUDO/distribution/FR [Accessed: 05 December 2022]
EPPO (European and Mediterranean Plant Protection Organization), 2009. Report of a Pest Risk Analysis for Bactrocera invadens. Available online: ttps://gd.eppo.int/taxon/DACUDO/documents
EPPO (European and Mediterranean Plant Protection Organization), 2016. EPPO Standard PM 7/129 (1) DNA barcoding as an identification tool for a number of regulated pests. EPPO Bulletin, 46, 501–537 .
EPPO (European and Mediterranean Plant Protection Organization), 2018. PM 7/134 (1) Dacus ciliatus. Bulletin OEPP/EPPO Bulletin 48 (3), 25–431 .
EPPO (European and Mediterranean Plant Protection Organization), 2022. EPPO Reporting Service no. 10 – 2022. Num. article: 2022/211. Update on the situation of Bactrocera dorsalis in Italy. Available online: https://gd.eppo.int/reporting/article-7442 [Accessed: 05 December 2022]
Eurostat, 2018. Regions in the European Union — Nomenclature of territorial units for statistics — NUTS 2016/EU-28, Edition 2018. https://ec.europa.eu/eurostat/web/products-manuals-and-guidelines/-/ks-gq-18-007
FAO (Food and Agriculture Organization of the United Nations), 2021a. ISPM (International Standards for Phytosanitary Measures) 6. Surveillance. FAO, Rome. 14 pp. Available online: https://www.ippc.int/en/publications/615/
FAO (Food and Agriculture Organization of the United Nations), 2021b. ISPM (International Standards for Phytosanitary Measures) 31. Methodologies for sampling of consignments. FAO, Rome. 21 pp. Available online: ttps://www.ippc.int/en/publications/588/
FAO (Food and Agriculture Organization of the United Nations), 2021c. Surveillance guide: A guide to understand the principal requirements of surveillance programmes for national plant protection organizations. Second edition. Rome, FAO on behalf of the Secretariat of the International Plant Protection Convention. Available online: https://www.fao.org/3/cb7139en/cb7139en.pdf
FAO (Food and Agriculture Organization), 2021d. Transient incursions of exotic Bactrocera species in Torres Strait. IPPC (International Plant Protection Convention) Official Report, No. AUS-61/5. FAO: Rome, Italy. Available online: https://www.ippc.int/fr/countries/australia/pestreports/2014/04/transient-incursions-of-exotic-bactrocera-species-in-torres-strait/ [Accessed: 05 December 2022]
FAO (Food and Agriculture Organization of the United Nations), 2018. International standards for phytosanitary measures, ISPM 26, establishment of pest free areas for fruit flies (Tephritidae). Food and Agriculture Organization of the United Nations, Rome, Italy.
FAO (Food and Agriculture Organization of the United Nations), 2019. International standards for phytosanitary measures, ISPM 27, Diagnostic protocols for regulated pests. DP 29: Bactrocera dorsalis. Food and Agriculture Organization of the United Nations, Rome, Italy.
Fletcher BS, 1987. The biology of Dacinae fruit flies. Annual Review of Entomology, 32, 115–144.
FDACS (Florida Department of Agriculture and Consumer Services), 2018. Bactrocera dorsalis, Oriental fruit fly (Hendel) (Tephritidae). FDACS-P-01884. Available online: https://www.freshfromflorida.com/content/download/81039/2340067/PEST_ALERT_Oriental_Fruit_Fly_-_Updated_June_2018.pdf
Froerer KM, Peck SL, McQuate GT, Vargas RI, Jang EB and McInnis DO, 2010. Long-Distance Movement of Bactrocera dorsalis (Diptera: Tephritidae) in Puna, Hawaii: How far can they go? American Entomologist, 56, 8–95.
Goergen G, Vayssières J-F, Gnanvossou D and Tindo M, 2011. Bactrocera invadens (Diptera: Tephritidae), a new invasive fruit fly pest for the Afrotropical Region: host plant range and distribution in West and Central Africa. Environmental Entomology, 40, 844–854.
Han P, Wang X, Niu CY, Dong YC, Zhu JQ and Desneux N, 2011. Population dynamics, phenology, and overwintering of Bactrocera dorsalis (Diptera: Tephritidae) in Hubei Province, China. Journal of pest science, 84, 289–295.
Koyama J, Teruya T and Tanaka K, 1984. Eradication of the oriental fruit fly (Diptera: Tephritidae) from the Okinawa Islands by a male annihilation method. Journal of Economic Entomology, 77, 468-472.
Kumar B and Agarwal M, 2005. Population fluctuation of Bactrocera dorsalis (Hendel) (Diptera: Tephritidae) based on Steiner type trap catches. Entomon, 30, 171–173.
Leblanc L, San Jose M, Barr N and Rubinoff D, 2015. A phylogenetic assessment of the polyphyletic nature and intraspecific color polymorphism in the Bactrocera dorsalis complex (Diptera, Tephritidae). Zookeys, 540, 39–367.
Lux SA, Copeland RS, White IM, Manrakhan A and Billah MK, 2003. A New Invasive Fruit Fly Species from the Bactrocera dorsalis (Hendel) Group Detected in East Africa. Insect Science and Its Application, 23, 355–361.
Manoukis NC, Jang EB and Dowell RV, 2017. Survivorship of male and female Bactrocera dorsalis in the field and the effect of male annihilation technique. Entomologia Experimentalis et Applicata, 162, 243–250.
Manrakhan A, 2016. Detection and monitoring of fruit flies in Africa. In: Ekesi S, Mohamed SA, and De Meyer M. (Eds). Fruit fly research and development in Africa-towards a sustainable management strategy to improve horticulture. Springer.Ndlela S, Mohamed S, Ekesi S, Ndegwa PN, and Ongamo GO, 016. Male annihilation technique using methyl eugenol for field suppression of Bactrocera dorsalis (Hendel) (Diptera: Tephritidae) on mango in Kenya. African Entomology, 24, 437–447.
NAPPO (North American Plant Protection Organisation), 2021. North American Plant Protection Organisation Phytosanitary Alert System. [Online] Available online: https://pestalerts.org/official-pest-report/bactrocera-dorsalis-oriental-fruit-fly-aphis-establishes-quarantine-santa [Accessed 24/01/2022 January 2022].
Nugnes F, Russo E, Viggiani G, and Bernardo U, 018. First record of an invasive fruit fly belonging to Bactrocera dorsalis complex (Diptera: Tephritidae) in Europe. Insects, 9, 1-11.
Odanga J, Mohamed S, Mwalusepo S, Olubayo F, Nyankanga R, Khamis F, Rwomushana I, Johansson T and Ekesi S, 2018. Spatial Distribution of Bactrocera dorsalis and Thaumatotibia leucotreta in Smallholder Avocado Orchards along Altitudinal Gradient of Taita Hills and Mount Kilimanjaro. Insects, 9, 71.
Papadopoulos NT, Plant RE and Carey JR, 2013. From trickle to flood: the large-scale, cryptic invasion of California by tropical fruit flies. Proceedings of the Royal Society B no. 208: 30131466.
Papadopoulos NT, Katsoyannos BI, Kouloussis NA, Hendrichs J, Carey JR and Heath RR, 2001. Early Detection and Population Monitoring of Ceratitis capitata. (Diptera: Tephritidae) in a Mixed-Fruit Orchard in Northern Greece. Journal of Economic Entomology, 94, 971–978.
Rwomushana I, Ekesi S, Gordon I & Ogol CK, 2008a Host plants and host plant preference studies for Bactrocera invadens (Diptera: Tephritidae) in Kenya, a new invasive fruit fly species in Africa. Annals of the Entomological Society of America 101: 331–340.
Rwomushana I, Ekesi S, Ogol C and Gordon I, 2008b. Effect of temperature on development and survival of immature stages of Bactrocera invadens (Diptera: Tephritidae). Journal of Applied Entomology, 132, 32–839.
Salum JK, Mwatawala MW, Kusolwa PM and Meyer MD, 2014. Demographic parameters of the two main fruit fly (Diptera: Tephritidae) species attacking mango in Central Tanzania. Journal of Applied Entomology, 138, 441–448.
Schutze MK, Aketarawong N, Amornsak W, Armstrong KF, Augustinos AA, Barr N and Bo W, 2015a. Synonymization of key pest species within the Bactrocera dorsalis species complex (Diptera: Tephritidae): Taxonomic changes based on a review of 20 years of the integrative morphological, molecular, cytogenetic, behavioural, and chemoecological data. Systematic Entomology, 40, 456–471.
Schutze M K, Mahmood K, Pavasovic ANA, Bo Z, Newman J, Clarke AR, Krosch MN and Cameron SL, 2015b. One and the same: integrative taxonomic evidence that Bactrocera invadens (Diptera: Tephritidae) is the same species as the Oriental fruit fly Bactrocera dorsalis. Systematic Entomology, 40(2), 472-486.
Schutze MK, Bourtzis K, Cameron SL, Clarke AR, De Meyer M, Hee AKW, Hendrichs J, Krosch MN and Mwatawala M, 2017a. Integrative taxonomy versus taxonomic authority without peer review: The case of the Oriental fruit fly, Bactrocera dorsalis (Tephritidae). Systematic Entomology, 42, 609–620.
Schutze MK, Virgilio M, Norrbom A, and Clarke AR 2017b. Tephritid integrative taxonomy: Where we are now, with a focus on the resolution of three tropical fruit fly species complexes. Annual review of entomology, 62, 147-164.
Shelly TE, Pahio E and Edu J, 2004. Synergistic and inhibitory interactions between methyl eugenol and cue lure influence trap catch of male fruit flies, Bactrocera dorsalis (Hendel) and B. cucurbitae (Diptera: Tephritidae). Florida Entomologist, 87, 481–487.
Shelly T, Nishimoto J, Diaz A, Leathers J, War M, Shoemaker R, Al-Zubaidy M and Joseph D, 2010. Capture probability of released males of two Bactrocera species (Diptera: Tephritidae) in detection traps in California. Journal of Economic Entomology, 103, 2042–2051.
Shelly TE, Lance DR, Tan KH, Suckling DM, Bloem K, Enkerlin W, Hoffman K, Barr K, Rodríguez R, Gomes PJ and Hendrichs J, 2017. To Repeat: Can Polyphagous Invasive Tephritid Pest Populations Remain Undetected For Years Under Favorable Climatic and Host Conditions? American Entomologist, 63, 24–231.
Steiner LF, 1957. Field evaluation of oriental fruit fly insecticides in Hawaii. Journal of Economic Entomology, 50, 16–24.
Stephens A, Kriticos D and Leriche A, 2007. The current and future potential geographical distribution of the oriental fruit fly, Bactrocera dorsalis (Diptera: Tephritidae). Bulletin of Entomological Research, 97, 69–378.
Theron CD, Manrakhan A and Weldon CW, 2017. Host use of the oriental fruit fly, Bactrocera dorsalis (Hendel) (Diptera: Tephritidae), in South Africa. Journal of Applied Entomology, 141, 810–816.
Vaníčková L, Nagy R, Pompeiano A and Kalinova B, 2017. Epicuticular chemistry reinforces the new taxonomic classification of the Bactrocera dorsalis species complex (Diptera: Tephritidae, Dacinae). PloS one, 12(9).
Vargas RI, Piñero JC, Mau RFL, Jang EB, Klungness LM, Mclnnis DO, Harris EB, McQuate GT, Bautista RC and Wong L, 2010a. Area-wide suppression of the Mediterranean fruit fly, Ceratitis capitata, and the Oriental fruit fly, Bactrocera dorsalis, in Kamuela, Hawaii. Journal of Insect Science, 0, 135.
Vargas RI, Mau RF, Stark JD, Piñero JC, Leblanc L and Souder SK, 2010b. Evaluation of methyl eugenol and cue-lure traps with solid lure and insecticide dispensers for fruit fly monitoring and male annihilation in the Hawaii areawide pest management program. Journal of economic entomology, 103, 409–415.
Vargas RI, Piñero JC and Leblanc L, 2015. An overview of pest species of Bactrocera fruit flies (Diptera: Tephritidae) and the integration of biopesticides with other biological approaches for their management with a focus on the Pacific region. Insects, 6, 97–318.
Weems HV, Heppner JB, Nation JL and Steck GJ, 1999. Oriental Fruit Fly, Bactrocera dorsalis (Hendel) (Insecta: Diptera: Tephritidae). EENY-083, EDIS, University of Florida, IFAS, Extension Service. Available online: http://edis.ifas.ufl.edu/pdffiles/IN/IN24000.pdf
White IM and Elson-Harris MM, 1992. Fruit flies of economic significance: their identification and bionomics. 601 pp. CAB International, Wallingford.
Qin Y, Krosch MN, Schutze MK, Wang X, Prabhakar CS and Susanto A, 2018. Population structure of a global agricultural pest, Bactrocera dorsalis (Diptera: Tephritidae). Evolutionary Applications, 11, 1990–2003.
Zhu YI and Qiu HT, 1989. The reestablishment of Dacus dorsalis Hendel (Diptera: Tephritidae) after flee eradication on Lanbay Island. Journal of Economic Entomology, 9, 217–230.